GALATEA
''Galatea'' is a vacuum test stand, see Figure 1 (left), especially designed to allow scans with alpha and beta particles. This is possible because there is no material between the sources and the detector. In the future, also the usage of a tunable LASER is envisioned.
The setup is currently configured to hold one segmented germanium detector with a read-out for up to 20 channels.
The detector is cooled via a cold finger submerged in an internal liquid nitrogen tank located inside the vacuum tank. The detector is shielded against IR radiation (emitted by the warm vacuum tank) by a cage with super-insulation foil and a copper hat with slits to insert sources. The hat can be turned with respect to the detector. One slit is on the side of the hat, the other on top. The sources are placed inside two collimators pointing through these slits. A system of three stages allows a complete scan of the mantle of a detector, see Figure 1 (right).
Galatea is a powerful high precision tool to investigate bulk and surface effects in germanium detectors.
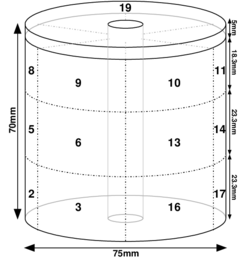
The first results published on GALATEA data were obtained by irradiating the 18+1-fold segmented true-coaxial detector Supersiegfried, see Figure 2, from the top with alpha particles. Supersiegfried is a detector with the main part of the cylinder segmented 3-fold in z in 6-fold in phi and additional 5mm thick top segment. This thin top segment was introduced to study events on a passivated end-plate of a true-coaxial detector. Alpha particles only penetrate about 25 micrometers into the detector. Thus, they deposit their energy very close to the surface, creating so-called electron-hole pairs, which are called charge carriers. The electrons drift towards the core of the detector and the holes towards the outer mantle. These drifting charges are not only observed in the top segment but also in the segments underneath, which allows detailed studies of the charge drift. It is also possible to show that charges are trapped close to the surface.
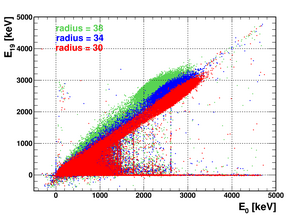
Underneath the passivation layer on the end-plate of Supersiegfried, a so called dead layer of around 100 micrometers was expected. This would have made the observation of alpha particles impossible. But they were observed, indicating that the actual dead layer was only about 10 micrometers thick. It was also observed that the energy registered in the core through the inside contact was not equal to the energy registered in the segment through the contacts on the top segment. This indicates that electrons (core) and holes (top segment) do not get trapped in a similar way. The larger the radius of the alpha event, the more likely it becomes that electrons get trapped on their way to the core. As a consequence, the core energy becomes smaller. The situation is reversed for holes drifting towards the outside of the detector. This is demonstrated in Figure 3.
Key publications
The GALATEA test-facility for high purity germanium detectors
Nucl.Instrum.Meth. A 782 (2015) 56
Alpha-event and surface characterisation in segmented true-coaxial HPGe detectors
Nucl. Instrum. Meth. A 858 (2017) 80-89
Measurement of the temperature dependence of pulse lengths in an n-type germanium detector
Eur. Phys. J. Appl. Phys. 56 (2011) 10104
Pulse shape simulation for segmented true-coaxial HPGe detectors
Eur. Phys. J. C 68, 609-618 (2010)
Neutron Interactions as Seen by A Segmented Germanium Detector
Eur. Phys. J. A 36, 139-149 (2008)
Characterization of the first true coaxial 18-fold segmented n-type prototype detector for the GERDA project Nucl.Instrum.Meth. A 577 (2007) 574
Key publications
The GALATEA test-facility for high purity germanium detectors
Nucl.Instrum.Meth. A 782 (2015) 56
Alpha-event and surface characterisation in segmented true-coaxial HPGe detectors
Nucl. Instrum. Meth. A 858 (2017) 80-89
Measurement of the temperature dependence of pulse lengths in an n-type germanium detector
Eur. Phys. J. Appl. Phys. 56 (2011) 10104
Pulse shape simulation for segmented true-coaxial HPGe detectors
Eur. Phys. J. C 68, 609-618 (2010)
Neutron Interactions as Seen by A Segmented Germanium Detector
Eur. Phys. J. A 36, 139-149 (2008)
Characterization of the first true coaxial 18-fold segmented n-type prototype detector for the GERDA project Nucl.Instrum.Meth. A 577 (2007) 574